Appears in
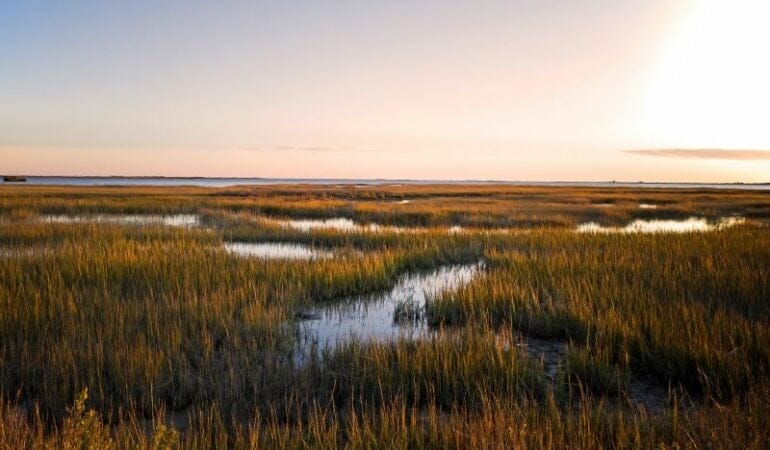
Nature’s Toolbox: Fungi, Marshes, and Other Unsung Climate Heroes
Climate change is no longer knocking—it kicked down the door this summer. Wildfires destroyed more than 33 million acres of forest in Canada’s worst-ever fire season. Vermont was flooded by a 100-year storm for the second time in 12 years, while a different deluge left five dead outside of Philadelphia. Temperatures in Phoenix crested 110ºF for 31 consecutive days, failing to dip below 90ºF at any time for more than two straight weeks. And as deadly bouts of flood, fire, and ferocious heat erupted all over the planet, we lived through the hottest day in recorded history—a global record that was promptly broken the very next day, and again the next—in what scientists say was likely the hottest month on Earth in 120,000 years.
Given the urgency of the climate crisis, every workable solution to limit further warming and to transition our economies off of fossil fuels deserves exploration. This dire situation demands technological advances, of course; indeed, technology has alleviated so much human suffering, it’s tempting to heave all our hopes squarely upon its back, like desperate sports fans expectantly looking to their team’s star player to pull off just one more spectacular play as the clock runs out.
But we can’t overlook the importance of allowing and encouraging nature to heal its own ecosystems as part of our climate strategy. And nature’s toolbox can be surprisingly effective.
Trees are often touted for the many small miracles they provide, especially in urban areas, as they cool streets, clean air, and reduce storm runoff while pulling carbon dioxide from the atmosphere. But how many people know that microscopic forest fungi process twice as much carbon as the United States emits each year? Or that a salt marsh can sequester 10 times as much carbon per acre as a forest? Or that restoring even a small fraction of bison populations across parts of the American prairie could help those grasslands absorb more carbon than all of Great Britain emits in a year?
These are not miracle cures for the climate crisis, of course; none of these tools will slow climate change on its own without a dramatic reduction of fossil fuel use. But all are surprisingly powerful, relatively simple, and low-risk strategies we could be using more often, in more places. After all, both an expensive cordless drill and a five-dollar screwdriver can help you build something—but only if you get to work using them.
Marsh Magic
Hundreds of millions of people around the world live near a salt marsh, or a similar coastal ecosystem of mangroves or seagrass. These seaside sanctuaries offer quiet beauty and attract abundant wildlife; they also absorb flood water and wave energy during storms, reducing damage to adjacent communities by up to 20 percent. But many people don’t realize that these unassuming tidal wetlands are also busy trapping carbon at an astonishing rate—10 to 40 times faster than a forest.
There are two reasons salt marshes, mangroves, and seagrass beds are such powerful carbon sinks. One is that their vegetation grows very quickly, says Hilary Stevens, coastal resilience manager at Restore America’s Estuaries. “There’s a lot of photosynthesis, a lot of pulling of carbon dioxide out of the atmosphere,” she explains.
But the real magic of the marsh is its salty, soggy soil. When that vegetation dies, it falls to the bottom of the marsh and gets buried in a network of roots and sediment, where it will remain indefinitely saturated with briny water. That anaerobic environment slows or even stops the decomposition process, allowing the carbon in the plants to stay stashed in the soil for hundreds or even thousands of years. This underwater vault is known as “blue carbon.”
While forests are also excellent at trapping carbon, Stevens says, they’re more likely to release it, through events ranging from wildfire to decomposition. But the carbon in marsh soil “can remain there for centuries if that area remains inundated and undisturbed.”
Of course, that’s a big “if” when there are humans around. The United States alone loses an estimated 80,000 acres of coastal wetlands each year due to a combination of development and sea level rise. Even many surviving marshes have been ditched and drained over the years, allowing air to reach the long-submerged soil, and turning powerful carbon sinks into leaky CO2 emitters.
“If you disturb an inundated soil, if you allow it to drain—whether that’s because you filled it, ditched it, diked it, drained it, converted it to agriculture, or paved it and put up a parking lot—all of that organic material is at risk of being rereleased into the atmosphere,” Stevens says. Centuries worth of carbon can then escape fairly quickly, so preventing further loss of healthy coastal wetlands is critical from a climate standpoint.
That’s a growing challenge as ocean levels rise more quickly. Salt marshes can sometimes migrate upland as rising seas encroach, says Cynthia Dittbrenner, director of coastal and natural resources at Massachusetts-based conservation organization The Trustees—but only if there’s room to do so, and the walls and roads of human development often make that impossible. And although they’re actually quite good at adapting to slowly rising seas—because a healthy salt marsh naturally builds in elevation each year as its grasses die off and accumulate on the bottom and daily tidal inflows deliver new sediment—scientists fear that natural process of accretion can’t keep up with the unnatural and accelerating rate of sea level rise driven by human-caused climate change.
What’s more, a lot of our remaining marshes aren’t particularly healthy.
In New England, for example, colonial farmers viewed salt marshes as a source of hay for livestock and horses, and set about draining them to ease harvesting. To this day, most of the region’s marshes are still ribboned with man-made ditches dug hundreds of years ago. Later, the long-neglected ditches clogged, creating pools of standing water that prompted 20th-century mosquito-prevention squads to dig them out once again. But a drained marsh doesn’t build elevation as it should; in fact, it sinks, because the organic matter in the soil starts decomposing more rapidly as it interacts with the air.
“A legacy of 300 years of us ditching the marsh has led to lowering the water table, and that marsh soil is now being exposed to oxygen,” Dittbrenner says. “It’s aerated, it’s decomposing quickly, and it’s actually sinking . . . so we have to heal the hydrology to fix that natural process.”
There are simple and cost-effective ways to restore ditch-drained salt marshes. One method, piloted by the US Fish and Wildlife Service, is to cut marsh grass along the edge of a ditch, rake the hay into the trench, and secure it to the bottom with twine and stakes. “When the tides come in, that hay slows the water down and encourages sediment to drop out,” Dittbrenner says, and that slowly refills the ditch. “If you do that over a series of three to four years, you’ve filled the ditch up, and now it can grow salt marsh hay,” and tidewater lingers longer as it naturally would.
The Trustees implemented that technique on 85 acres in the Great Marsh north of Boston, and the results were so promising that the organization secured funding—and hard-won permits—to expand the restoration effort across all of the 1,400 acres it manages there.
Another opportunity for marsh restoration is in places where a road or bridge has cut off part of a marsh from incoming seawater. “The area upstream of that essentially becomes freshwater, because it’s not getting enough tidal influence,” Stevens says. Soil inundated with freshwater is still slow to release carbon dioxide, she notes, but it does emit a lot of methane—a much more potent greenhouse gas—because it hosts a different set of microbes not found in brackish or saltwater. “If you can restore tidal flow to those areas, there’s a massive carbon benefit to that.”
One such effort underway is the Herring River Restoration Project in Wellfleet, Massachusetts, where a dike built in 1909 cut off tidal flow to what had been a stable estuary for 2,000 years. A new bridge with large tide gates will allow ocean water to return—gradually, at first—along with herring and other fish, tidal sediment, and native saltwater seagrasses. The project will return 677 acres of freshwater wetland to salt marsh, which the US Geological Survey calculates will reduce emissions by an equivalent of nearly 3,000 tons of carbon per year.
With less room to migrate, smaller, narrow “fringe” marshes are more at risk from rising seas. But they can “attenuate quite a bit of wave energy,” Dittbrenner says, making them useful storm defenses for coastal communities, and they can be protected—or even created—with simple, natural methods. Installing coir logs (made of coconut husk fiber) or mesh bags stuffed with oyster shells a few meters offshore, for example, can help protect and grow the marsh behind it. “It slows the wave energy so much that it allows sediment to build up,” she says, until grass can grow on it. In one project, Dittbrenner says, researchers were able to extend a stretch of fringe marsh 10 feet further into the water in less than a year using old lobster traps to slow the waves and capture sediment.
Stevens is now working on a project in the Gulf Coast that uses recycled oyster shells from restaurants to build artificial reefs, creating new habitat for live oysters. In addition to increasing food security for the community (oysters, she notes, are one of the most climate-friendly ways to grow protein, requiring no irrigation, fertilizer, or feed), the reefs create a breakwater to stabilize the shoreline and protect adjacent communities.
But halting the continued loss of coastal wetlands would have the biggest climate impact of all. “We would love to see better protection for existing blue carbon ecosystems,” Stevens says, along with a more coordinated government approach—two pillars of Restore America’s Estuaries’ Blue Carbon National Action Plan.
At the same time, Stevens says, there’s a need for permitting reform, so groups like RAE and The Trustees can more easily restore degraded marshes; it can be difficult to reuse dredged sediment, for example, even though it’s a key ingredient to help sinking marshes. “Some of those regulations, because of the way they’re worded, actually inhibit restoration, because they make it so difficult to operate in the coastal zone,” she says. Such rules were put in place with the best of intentions, she adds, but that was decades ago. “And we’ve learned a lot since then.”
Forest-Feeding Fungi
Neither plant nor animal (though more closely related to the latter), fungi are their own biological kingdom, comprising about 3.5 million different species. Microscopic fungi are everywhere—all over our bodies, on plants, in the air we breathe—and without them, says Jennifer Bhatnagar, associate professor of biology at Boston University, most of the biological processes on earth would cease. They’re especially important in forests.
“One of the main roles of those fungi in forests is to decompose dead plant leaves, roots, and other plant parts, and other dead microorganisms, and most of that activity happens in the soil,” she says. In doing so, they release elements like nitrogen, phosphorus, and sulfur back into the soil in a form that plants can use.
But there’s a group that’s particularly crucial to forest health: mycorrhizal fungi, which live on the roots of plants in one of the oldest symbiotic relationships found in nature.
When a mycorrhizal fungus colonizes a plant by growing on or inside of its root cells, the plant will send up to 30 percent of its carbon—in the form of sugar, produced through photosynthesis—from its leaves down to its roots to feed the fungus. To return the favor, “the fungus will use that carbon to extend out into the soil and absorb those nutrients that are being decomposed by other fungi,” Bhatnagar says, and give them to the plant. The carbon ends up feeding not just the fungus, but also nearby microbes, which help sequester it in the soil.
“This is the main way that plant carbon gets from above ground to below ground on the surface of the earth,” Bhatnagar says. “It’s a really important way that we’re able to take carbon out of the atmosphere and put it into the earth—and it can remain there for quite a long time.”
A study published in June estimated that an astounding 13.12 gigatons of carbon fixed by plants each year is allocated to mycorrhizal fungi, at least temporarily. It’s not yet known how much of that carbon is retained in the soil long-term, but even half of that would represent more than the annual carbon-equivalent emissions of the United States—and the researchers suggested that fungi could be essential to reaching net zero.
Mycorrhizal fungi networks can help boost a forest’s carbon intake above ground as well. Ecologist Colin Averill, lead scientist at ETH Zurich’s Crowther Lab and the founder of the carbon removal start-up Funga, says it’s helpful to think about the microbial environment of soil the way we conceive of the human gut biome. “Each of us has this incredibly biodiverse community of bacteria in our gut, and this has profound implications for our health,” Averill says—and a forest is no different.
To learn what a healthy forest microbiome looks like, he and his team compared soil samples from hundreds of locations across Europe where foresters had been tracking trees for decades. They found that the mix of fungi living on the tree roots in the sampled forests was linked to a threefold variation in how fast the trees grew. Put another way, Averill says, “You could have two pine forests in Central Europe sitting side by side, experiencing the same climate, growing in the same soils. But if one of them has the right community of fungi on its roots, it can be growing up to three times as fast as that adjacent forest,” and removing more carbon from the atmosphere.
This can have a particularly profound impact on the reforestation of former agricultural land or other degraded landscapes, where, after decades of farming, grazing, or mining, Averill says, “The microbes that live in that soil no longer look anything like the microbes in a forest.”
Averill partnered with a nonprofit in Wales that was reforesting an abandoned sheep pasture to conduct an experiment, adding a handful of soil from a healthy forest to some of the saplings as they were planted. “It’s a very low-tech procedure,” he says. “But it’s not just any dirt. It’s dirt from a forest that our analyses identified as harboring intact wild, biodiverse, high-performing fungal communities. And the early results there show we can accelerate forest regeneration by 30 to 70 percent if we co-reintroduce the below-ground microbiology.”
Similar experiments around the world that introduced healthy microbial networks to degraded forest or grassland soil have shown a 64 percent average increase in biomass growth, Averill says—though the results vary widely. “Some places are unresponsive, some are incredibly responsive,” he says. “But basically what we’re learning is that there’s something special about wild microbiology that can be lost, and it can have this enormous effect if you reintroduce it.”
Letting Wildlife Go Wild
For another way to accelerate forest growth and carbon uptake, we turn to a different biological kingdom: animalia.
A 2023 study led by Yale ecology professor Oswald J. Schmitz found that protecting and restoring populations of animal species can supercharge the carbon capture capabilities of their respective ecosystems. This can enhance the total amount of CO2 naturally absorbed and stored by as much as 6.41 gigatons per year worldwide—or more than 14 trillion pounds of CO2.
“People assume that because animals are rare in ecosystems, they don’t matter to ecosystem functioning,” Schmitz says. But the idea of “trophic cascades”—in which predators, by preying on herbivores, have a ripple effect on vegetation—made him think otherwise. “If predators can have a profound effect on plants, and we know herbivores can have a profound effect on plants, then surely they should also have an effect on carbon cycling and nutrient cycling.”
They do, and the carbon impact of healthy wildlife populations can be tremendous in all kinds of ecosystems.
Endangered forest elephants in central Africa, for example, spread the seeds of trees and woody plants, and trample and devour vegetative undergrowth, helping carbon-dense overstory trees grow faster and bigger. Restoring wild elephant populations within the region’s 79 national parks and protected areas—about 537,000 square kilometers of tropical rainforest—could help capture an estimated 13 megatons of additional CO2 per year, or 13 million metric tons.
In the ocean, migrating marine fish eat algae near the surface, and their fecal matter drops to the ocean floor or nourishes photosynthesizing phytoplankton. Fish also help the ocean lock up carbon as they rid their bodies of excess salt through the production of calcite, a form of calcium carbonate. “Calcite is a way of binding up salt,” Schmitz says, “but it’s also a carbon-based unit.” The hard pellets sink to the ocean bottom, and don’t break down easily. Marine fish currently help the oceans absorb 5.5 gigatons of CO2 annually—without getting explicit credit for it—and Schmitz says overharvesting fish or catching them in deeper waters could jeopardize that enormous underwater carbon vault.
Predators like sea otters, meanwhile, help carbon-absorbent kelp forests thrive by keeping seaweed-munching sea urchins in check. Gray wolves and sharks create similar trophic cascades in boreal forests and coral reefs, where they keep the populations of their smaller herbaceous prey in balance.
In the arctic, organic matter in the ground doesn’t decay and release methane as long as the permafrost stays frozen. Caribou and muskoxen help ensure that by trampling arctic snowpack, creating a cold crust of compressed snow that forms an insulative barrier over the permafrost. Meanwhile, just by eating and trampling shrubs, they help the snow reflect more solar radiation. “If the animals aren’t there, the shrubs grow above the snowpack level, the sun shines on the vegetation and, especially in the spring, that vegetation holds the solar radiation,” Schmitz says. “It doesn’t reflect it the way snow would, and it warms up the soil a lot faster.”
And in North America, where white settlers all but wiped out the more than 30 million bison that once roamed the prairies, just 2 percent of that animal’s one-time numbers remain, confined to about 1 percent of its historical range. Because heavy herds of grazing bison help grasslands retain carbon in the soil, restoring their numbers across even a small fraction of the landscape—less than 16 percent of a handful of prairies where human conflict would be minimal—could help those ecosystems store an additional 595 megatons of CO2 annually, the study found.
That’s more than 10 percent of all the CO2 emitted by the United States in 2021. “We could restore up to 2 million bison in parts of the prairie states where they’re going to have very little conflict with people, and in doing that, you will be able to take up enough carbon to offset all of the prairie states’ fossil fuel emissions,” Schmitz explains.
These findings could have a meaningful impact on land and marine conservation efforts, says Jim Levitt, director of the International Land Conservation Network (ILCN) at the Lincoln Institute. “This is not your everyday piece of natural climate solution research,” says Levitt, who was not involved in the study. “I think this is a major insight.”
For one thing, it points to the need for larger, more interconnected wild spaces. “It’s not just land protection, it’s also stewardship across big corridors, large landscape conservation,” Levitt says. Animals need huge swaths of functionally intact ecosystems to recover their historical numbers and species diversity, but they can rebound rapidly given the right conditions.
“If you give nature a chance to reestablish itself, it’s really efficient at doing so,” Levitt says, noting that many US National Forests were once abandoned lands denuded of their timber. Now those swaths of forest are essential tools for absorbing atmospheric carbon.
“Not only do the trees sequester carbon, but the soil, the animals, the insect life, and the mycorrhizal networks under the ground, they’re all sequestering carbon, and they all depend on a healthy chain of trophic networks,” Levitt says. “So there is utility, even related to the survival of our species, in having wild animals on open space. It’s not just beautiful, it keeps the carbon cycle in tune.”
As a resource hub connecting private and civic conservation groups across cultural and political boundaries, Levitt says ILCN has an important role to play in supporting the establishment of the type of linked, protected environments that promote greater biodiversity. “You really need large, interconnected, protected spaces to get to truly rich ecosystems,” he says. “And what networks can do is make land conservation contagious sociologically—meaning, if your next-door neighbor has conserved his property, you’re more likely to do the same thing.” ILCN also supports the global 30×30 effort, an agreement among more than 190 countries to work toward protecting 30 percent of the world’s land and oceans by 2030.
With that ambitious global conservation goal in mind, Schmitz contends that the recent study demands a shift in perspective, and an embrace of more dynamic landscapes. “We can’t just do it in parks and protected areas, there just isn’t enough [protected space],” Schmitz says. “So we actually have to think about working landscapes.”
And that’s where human-wildlife conflict can occur, as wild animals trample crops, for example. To ease that tension, Schmitz suggests paying landowners for lost livelihoods as well as for the carbon they’re offsetting. “If we’re going to ask people to live with these animals, we should at least compensate them . . . but also we should inspire them to think differently about being stewards of their lands,” he adds. “Instead of having cattle ranchers in the western prairies, maybe there are some people who’d think of themselves better as carbon ranchers, who are willing to bring bison back, and we should actually pay them for the service that provides.”
Jon Gorey is a staff writer for the Lincoln Institute of Land Policy.
Lead image: Coastal salt marsh in Virginia. Credit: McKinneMike via iStock/Getty Images Plus.